Dr M Carmo Vasconcelos
As vineyards age and succumb to trunk disease, there is an urgent need for information to support replanting decision-making. The selection of rootstock is one of the most important decisions in the development of a vineyard. To address this issue, BRI recently started a rootstock research programme. A new research trial was planted in October 2022 in the Wairau Valley of Marlborough. It includes 14 rootstocks combined with three levels of irrigation, with the goal of identifying rootstocks that will prepare us for a warmer and drier future.
The domesticated grapevine is a composite of two genomes. The root system of the vine, or the connection between the soil environment and the plant, is represented by the rootstock genotype. Rootstocks are crucial for storing nutrients and carbon assimilates and absorbing water and minerals. The grafting point designates the junction between the root (below-ground organs, i.e. rootstocks) and the shoot (above-ground organs, such as those of V. vinifera).
Rootstocks were originally selected for pest resistance, ease of rooting, grafting, propagation, and limestone tolerance. By providing the interface between the soil and the scion, rootstocks also impact nutrient and water uptake and many aspects of scion physiology via hydraulic and chemical signalling.
Anthropogenic climate change has impacted viticulture in almost all wine regions in recent decades, primarily due to rising temperatures, changing precipitation patterns, and an increase in the frequency of extreme events (Pörtner et al., 2022), affecting grape yield and quality. Drought, flooding, and soil erosion are only a few indirect effects of climate change that limit productivity and alter grape composition. Adaptation and mitigation are two strategies for reducing and controlling the impact of climate change.
New adaptation strategies are required to deal with climate change, and using more efficient rootstocks is a sustainable solution.
Higher global air temperature and intensity of climatic anomalies are projected to increase evaporative demand (Pörtner et al., 2022). These effects could be mitigated by increasing transpiration rates and lowering leaf temperature.
The water footprint of agriculture is under increased scrutiny, and industries need to be prepared for future restrictions on water available for irrigation. Optimising water use for vineyards by increasing water use efficiency (WUE) is critical to ensure viticulture’s long-term viability. Rootstocks have been reported to alter the gas-exchange performance of the scion and regulate water use efficiency (Barrios-Masias et al., 2015; Bartlett et al., 2022; Berdeja et al., 2014; Candolfi-Vasconcelos et al., 1994; Carbonneau, 1985; Cuneo et al., 2021; Dargie et al., 2014; de Souza et al., 2022; Lucini et al., 2020; Marguerit et al., 2012; Reingwirtz et al., 2021; Sampaio, 2007; Tsegay et al., 2014; Yildirim et al., 2018; Zhang et al., 2016; Zombardo et al., 2020).
Understanding geographic origins and characteristics of rootstocks
Interspecific hybrids of the American species Vitis riparia, Vitis rupestris, and Vitis berlandieri make up the majority of rootstock types. Knowledge of the geographic origins and characteristics of the parent species helps in understanding the potential attributes of their crosses.
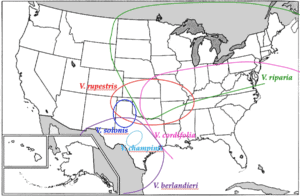
Figure 1. Geographical origin of the main American Vitis species used as rootstocks
Wild Vitis riparia spreads over the largest geographic area, from the centre of Canada to Texas and Louisiana in the south and extends to eastern Canada and the American states bordering the Atlantic (Figure 1). It grows on riverbanks in moist, fertile soils. The root system is shallow growing. It has high resistance to phylloxera, resistance to humidity, has a short vegetative cycle and is adapted to cold climates. It has low to moderate vigour and low tolerance to limestone. It grafts well (Galet, 1988; Pongrácz, 1983). Vitis riparia Gloire de Montpellier is a widespread selection used as a devigourating rootstock in fertile soils.
Vitis rupestris is native to the South of the United States. It is a shrub rather than a vine. It is found in the wild on gravelly banks of mountain streams. It has high resistance to phylloxera, is resistant to drought in deep soils with penetrable subsoils, has a very long vegetative cycle, and is very vigorous. The root system is very deep growing. It roots easily and grafts well. It is sensitive to lime-induced chlorosis and does not tolerate wet feet (Galet, 1988; Pongrácz, 1983). Rupestris du Lot is a variety used in warm climates with long growing seasons.
Vitis berlandieri is native to the limestone hills of southwest Texas and grows in northern Mexico. It has an excellent grafting affinity with V. vinifera. This species is not used as a rootstock because its cuttings are very difficult to root. That is why it has been crossed with easy-rooting species such as V. riparia and V. rupestris. V. berlandieri was originally selected for its resistance to lime-induced chlorosis. It is a species best suited to hot climates and has a long vegetative cycle. It is very vigorous and very drought tolerant (Galet, 1988; Pongrácz, 1983).
There have been several long-term rootstock trials conducted by Plant and Food Research comparing the performance of Sauvignon blanc grafted to five rootstocks (3309 C, 101-14 Mgt, Schwarzmann, Teleki 5C, Kobber 125 AA) (Neal et al., 2014). Commercial rootstock trials have also generated valuable information on yield performance and fruit composition. The objectives of those studies did not include rootstock’s impact on the water relations of the scion. We propose focusing this study on rootstocks’ performance under limited water availability. Rootstocks that can confer improved water efficiency will contribute to the industry’s resilience.
The main rootstocks in New Zealand vineyards are interspecific hybrids of V. riparia and V. rupestris. As a group, they have low to moderate drought tolerance and confer low to moderate vigour to the scion (Shaffer et al., 2004). Figure 2 shows the parentage of the most common rootstocks.
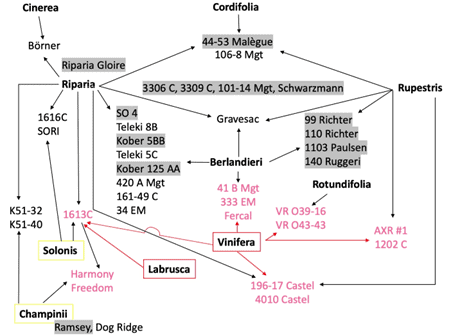
Figure 2. Parentage of internationally available rootstocks according to their breeders. The rootstocks used in the BRI trial are highlighted in grey.
Crosses of berlandieri X riparia and berlandieri X rupestris have been shown to have a higher tolerance to drought than the riparia X rupestris crosses. They also confer moderate to high vigour to the scion and are potentially better suited to a warmer and drier climate resulting from global warming (Sampaio, 2007).
One of the main issues limiting plant productivity is the availability of water; hence one important strategy for improving agrosystems is the development of crop types that use water more efficiently (Condon et al., 2004).
To maximise yield, organic and inorganic assimilates must be preferentially allocated to the harvested organ, the grapes in viticulture. Ravaz (1906) proposed the use of pruning weight as a proxy for canopy size and vegetative growth and popularised the use of the ratio of yield to pruning weight as an estimate of the balance between reproductive and vegetative growth of grapevines. Based on his observations, the ideal values for what is now called Ravaz Index (RI) range between five and seven. Sauvignon blanc grown in Marlborough produce yearly an enormous quantity of wood, 90% of which is removed with winter pruning and displays a RI between one and four. This low ratio may be related to the need to reinforce shoot tissues with thick cell walls to enable the vine to withstand high winds. However, improving this ratio should be one of the criteria for rootstock selection.
Besides tolerance to phylloxera, rootstocks also provide some protection against other pathogens such as nematodes (Edwards, 1989; Ferris et al., 2012; McKenry & Anwar, 2006; Pinkerton et al., 2005; Smith et al., 2018; Téliz et al., 2007; Wallis, 2020), mealybugs (Naegele et al., 2020) and may be more or less tolerant of trunk disease pathogens (Gramaje et al., 2014).
Literature cited
Barrios-Masias, F., Knipfer, T., & McElrone, A. (2015). Differential responses of grapevine rootstocks to water stress are associated with adjustments in fine root hydraulic physiology and suberization. Journal of Experimental Botany, 66(19), 6069-6078.
Bartlett, M., Sinclair, G., Fontanesi, G., Knipfer, T., Walker, M., & McElrone, A. (2022). Root pressure–volume curve traits capture rootstock drought tolerance. Annals of Botany, 129(4), 389-402.
Berdeja, M., Hilbert, G., Dai, Z. W., Lafontaine, M., Stoll, M., Schultz, H. R., & Delrot, S. (2014). Effect of water stress and rootstock genotype on Pinot Noir berry composition. Australian Journal of Grape and Wine Research, 20(3), 409-421.
Candolfi-Vasconcelos, M. C., Koblet, W., Howell, G., & Zweifel, W. (1994). Influence of defoliation, rootstock, training system, and leaf position on gas exchange of Pinot noir grapevines. American Journal of Enology and Viticulture, 45(2), 173-180.
Carbonneau, A. (1985). The early selection of grapevine rootstocks for resistance to drought conditions. Am. J. Enol. Vitic., 36, 195-198.
Condon, A. G., Richards, R., Rebetzke, G., & Farquhar, G. (2004). Breeding for high water-use efficiency. Journal of Experimental Botany, 55(407), 2447-2460.
Cuneo, I. F., Barrios-Masias, F., Knipfer, T., Uretsky, J., Reyes, C., Lenain, P., Brodersen, C. R., Walker, M. A., & McElrone, A. J. (2021). Differences in grapevine rootstock sensitivity and recovery from drought are linked to fine root cortical lacunae and root tip function [https://doi.org/10.1111/nph.16542]. New Phytologist, 229(1), 272-283. https://doi.org/https://doi.org/10.1111/nph.16542
Dargie, T., Dor, A., Manuel, A., & Molly, C. (2014). Responses of grapevine rootstocks to drought stress. International Journal of Plant Physiology and Biochemistry, 6(1), 1-6.
de Souza, C. R., Gindro, K., Verdenal, T., Spring, J.-L., Spangenberg, J. E., & Zufferey, V. (2022). Water deficit responses of field-grown Pinot noir mediated by rootstock genotypes in a cool climate region. Oeno one, 56(2), 136-148.
Edwards, M. (1989). Resistance and tolerance of grapevine rootstocks to plant-parasitic nematodes in vineyards in northeast Victoria Australia. Aust. J. Exp. Agric, 29, 129-132. https://doi.org/10.1071/EA9890129
Ferris, H., Zheng, L., & Walker, M. (2012). Resistance of grape rootstocks to plant-parasitic nematodes. Journal of Nematology, 44(4), 377-386.
Galet, P. (1988). Cépages et vignobles de France, Tome I: Les vignes américaines. 2e. Edition. Déhan, Montpellier.
Gramaje, D., Alaniz, S., Abad-Campos, P., García-Jiménez, J., & Armengol, J. (2014). Evaluation of grapevine rootstocks against soilborne pathogens associated with trunk diseases. I International Symposium on Grapevine Roots 1136,
Lucini, L., Miras-Moreno, B., Busconi, M., Marocco, A., Gatti, M., & Poni, S. (2020). Molecular basis of rootstock-related tolerance to water deficit in Vitis vinifera L. cv. Sangiovese: A physiological and metabolomic combined approach. Plant Science, 299, 110600. https://doi.org/https://doi.org/10.1016/j.plantsci.2020.110600
Marguerit, E., Brendel, O., Lebon, E., Van Leeuwen, C., & Ollat, N. (2012). Rootstock control of scion transpiration and its acclimation to water deficit are controlled by different genes. New Phytologist, 194(2), 416-429.
McKenry, M., & Anwar, S. A. (2006). Nematode and grape rootstock interactions including an improved understanding of tolerance. Journal of Nematology, 38(3), 312.
Naegele, R. P., Cousins, P., & Daane, K. M. (2020). Identification of Vitis cultivars, rootstocks, and species expressing resistance to a Planococcus mealybug. Insects, 11(2), 86.
Neal, S., Friend, A., Trought, M., & McLachlan, A. (2014). The performance of’Sauvignon Blanc’on five grapevine rootstocks in a Marlborough vineyard. XXIX International Horticultural Congress on Horticulture: Sustaining Lives, Livelihoods and Landscapes (IHC2014): IV 1115,
Pinkerton, J. N., Vasconcelos, M. C., Sampaio, T. L., & Shaffer, R. G. (2005). Reaction of grape rootstocks to ring nematode Mesocriconema xenoplax. Am J Enol Vitic, 56(4), 377-385.
Pongrácz, D. P. (1983). Rootstocks for grapevines. David Philip, Cape Town.
Pörtner, H. O., Roberts, D. C., Adams, H., Adler, C., Aldunce, P., Ali, E., Begum, R. A., Betts, R., Kerr, R. B., & Biesbroek, R. (2022). Climate change 2022: impacts, adaptation and vulnerability. Working Group II Contribution to the Sixth Assessment Report of the Intergovernmental Panel on Climate Change.
Ravaz, L. (1906). Influence de la surproduction sur la végétation de la vigne. Coulet.
Reingwirtz, I., Uretsky, J., Cuneo, I. F., Knipfer, T., Reyes, C., Walker, M. A., & McElrone, A. J. (2021). Inherent and stress-induced responses of fine root morphology and anatomy in commercial grapevine rootstocks with contrasting drought resistance. Plants, 10(6), 1121.
Riaz, S., Pap, D., Uretsky, J., Laucou, V., Boursiquot, J.-M., Kocsis, L., & Andrew Walker, M. (2019). Genetic diversity and parentage analysis of grape rootstocks. Theoretical and Applied Genetics, 132(6), 1847-1860.
Sampaio, T. (2007). Using rootstocks to manipulate vine physiological performance and mediate changes in fruit and wine composition Oregon State University]. Corvallis.
Shaffer, R. G., Sampalo, T. L., Pinkerton, J., & Vasconcelos, M. C. (2004). Grapevine rootstocks for Oregon vineyards [Technical Report]. Extension Service, Oregon State University. https://doi.org/http://localhost/files/gf06g291g
Smith, H. M., Smith, B. P., Morales, N. B., Moskwa, S., Clingeleffer, P. R., & Thomas, M. R. (2018). SNP markers tightly linked to root knot nematode resistance in grapevine (Vitis cinerea) identified by a genotyping-by-sequencing approach followed by Sequenom MassARRAY validation. PLoS One, 13(2), e0193121.
Téliz, D., Landa, B. B., Rapoport, H. F., Camacho, F. P., Jiménez-Díaz, R. M., & Castillo, P. (2007). Plant-parasitic nematodes infecting grapevine in southern Spain and susceptible reaction to root-knot nematodes of rootstocks reported as moderately resistant. Plant Disease, 91(9), 1147-1154.
Tsegay, D., Amsalem, D., Almeida, M., & Crandles, M. (2014). Responses of grapevine rootstocks to drought stress. International Journal of Plant Physiology and Biochemistry, 6(1), 1-6.
Wallis, C. M. (2020). Grapevine (Vitis spp.) rootstock stilbenoid associations with host resistance to and induction by root knot nematodes, Meloidogyne incognita. BMC Research Notes, 13(1), 1-7.
Yildirim, K., Yagci, A., Sucu, S., & Tunc, S. (2018). Responses of grapevine rootstocks to drought through altered root system architecture and root transcriptomic regulations. Plant Physiol Biochem, 127, 256-268. https://doi.org/10.1016/j.plaphy.2018.03.034
Zhang, L., Marguerit, E., Rossdeutsch, L., Ollat, N., & Gambetta, G. A. (2016). The influence of grapevine rootstocks on scion growth and drought resistance. Theoretical and Experimental Plant Physiology, 28(2), 143-157.
Zombardo, A., Mica, E., Puccioni, S., Perria, R., Valentini, P., Mattii, G. B., Cattivelli, L., & Storchi, P. (2020). Berry quality of grapevine under water stress as affected by rootstock–Scion interactions through gene expression regulation. Agronomy, 10(5), 680.